UTIG’s postdoc on discovering a new kind of tectonic behavior.
GEOPHYSICS Q&A
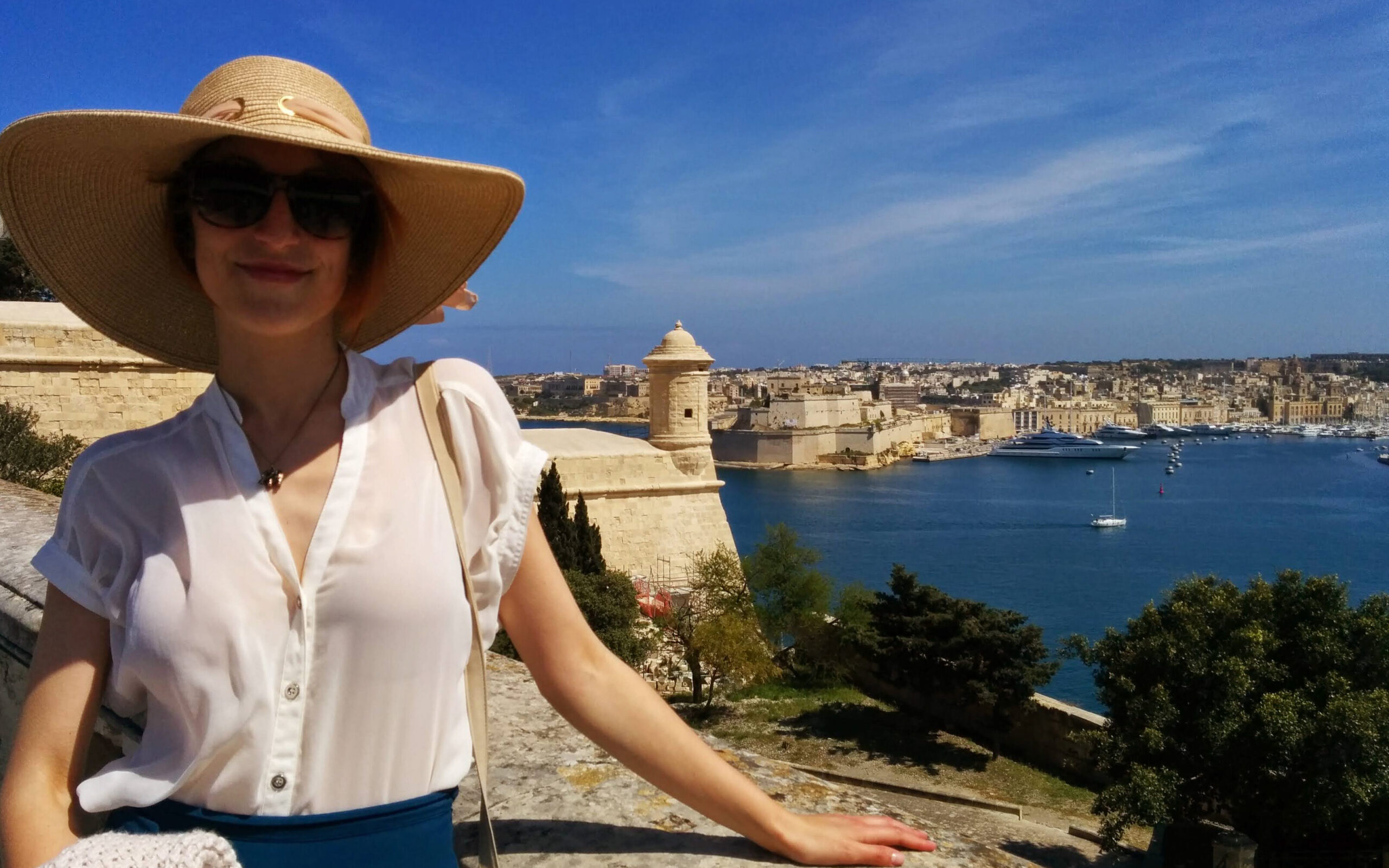
Before joining the University of Texas Institute for Geophysics, Antoniette Greta Grima discovered a previously unknown tectonic plate behavior that she called ‘slab orphaning.’
The process, which occurs 660 kilometers (410 miles) under the Earth’s surface, describes the way tectonic plates — or slabs — shed fragments into the Earth’s lower mantle. Her theory explained why the Earth’s deep interior is littered with these fragments and shook up long held views about the fate of some tectonic plates.
A native of the central Mediterranean island nation of Malta, Grima has been fascinated by tectonics since she was 11 years old. After graduating from the University of Malta, she went to graduate school at University College London (UCL) where she investigated the tectonic evolution of the Ionian basin — an unusually ancient and seismically active piece of oceanic crust that sits between southern Italy, Albania, and Greece. This began an interest in deep Earth processes that would eventually lead to the discovery of slab orphaning.
Now, as a postdoctoral fellow, Grima is continuing her research at UTIG where she is drawing on the power of Frontera, the largest academic supercomputer in the world (housed just next door at the Texas Advanced Computing Center), to investigate how forces in the Earth’s lower mantle might shape the surface.
She has also begun a new project funded by UTIG’s Blue Skies initiative to explore subduction on Jupiter’s moon, Europa, the only place in our solar system other than Earth to show clear signs of active plate tectonic-like behavior on the surface of its icy shell.
Below, Grima tells the story of her discovery, why she nearly wrote it off as a calculation error, and the new discoveries she’s working on at UTIG.
What is slab orphaning and how does it differ from the currently held view of what happens to plate tectonics at depth?
Slab orphaning shows how subducting tectonic plates — called slabs — break at the top of the lower mantle, which is about 660 kilometers depth.
Imagine the layers of the Earth: the core, mantle, and tectonic plates (which include the crust and some of the upper mantle). Tectonic plates are constantly moving over and under each other. When a plate is subducting, it is being pulled deeper into the mantle.
When a slab reaches the top of the lower mantle, it breaks. The bit that breaks is now the slab orphan. The rest of the slab — the slab parent — keeps on subducting but now it’s flattened on top of the lower mantle.
This was quite a breakthrough because before, the assumption was always that slabs break close to the surface and slowly sink through the mantle over hundreds of millions of years. In that scenario, subduction has to stop, which means you have to change the direction plates move everywhere else. With slab orphaning, subduction continues uninterrupted without any chaotic reorganization.
This is very important for understanding what our planet’s geography, oceans, and even climate looked like in the past and how it evolved to the present day.
Slab orphaning also explains why we see two types of slabs, those that are flat at 660 kilometers depth and those that sink to 1,000 kilometers depth and deeper. It shows the two are linked: a slab that has orphaned is one that reached 1,000 kilometers depth, then orphaned, leaving the parent slab to flatten at 660 kilometers depth.
Credit: Antoniette Greta Grima
What was your reaction when you made your discovery?
My first thought was “Oh my god, I think I broke my models.” I went back and reran the models several times, and triple, quadruple checked everything, but it was still there.
I had never seen anything like it before, so I genuinely thought it was a mistake. I changed the initial conditions and tried different setups, but it was still there. Whatever I did, I couldn’t make it go away! So I thought “Okay, maybe I should look into this a little bit more.”
Why were you so surprised?
As geoscientists, when we’re trying to explain how subduction works on Earth, we always talk about two plates meeting: one of them is lighter and stronger, and the other one is denser and colder and sinks under the other. Then, the subducted plate either flattens or penetrates the lower mantle. In this case, I was seeing a slab that was penetrating the lower mantle, it was breaking at the top of the lower mantle, and then it was flattening; so it was doing everything.
The whole concept that a slab can sink to the lower mantle and then, Bam!, break into two and continue on its own sweet way as if nothing happened was completely new to me. I had never seen the idea that a slab could or should break deep within the mantle and I had never seen it discussed in the literature before.
Why were you hunting for a new behavior in tectonic plates?
Our original plan was to investigate how the relationship between the slab and the mantle changes with depth. We were exploring a new viscosity profile in our subduction model when we saw a slab breaking at 660 kilometers depth. So it was a little bit accidental science!
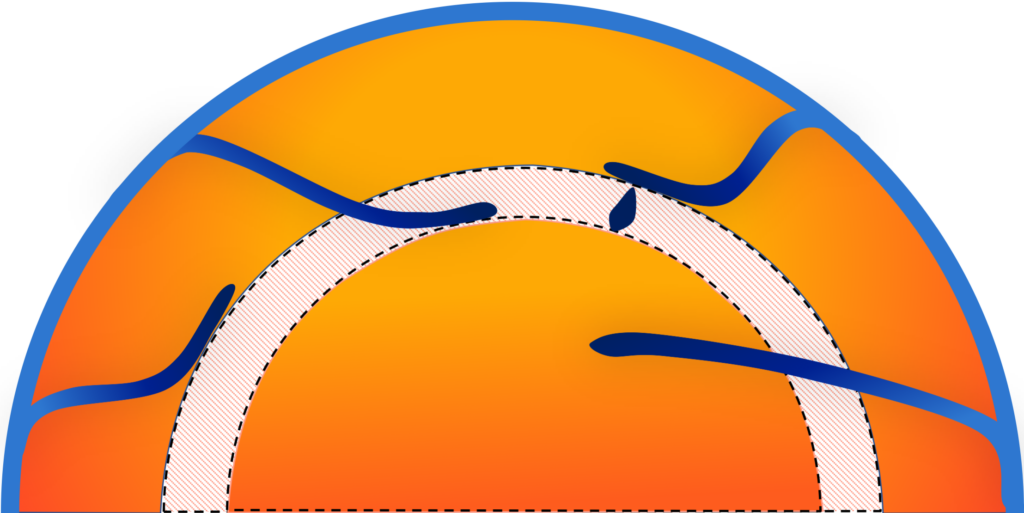
You said you were exploring a new viscosity structure for the Earth: are rocks molten at this depth?
Even at this depth, rocks are solid, but over millions of years (which is the timeframe we are interested in) the Earth’s mantle can behave very differently. Imagine the upper mantle as having a consistency like honey, and further down in the lower mantle because of increases in pressure and temperature it becomes stickier, like treacle (molasses).
The viscosity structure we were exploring in our model has three layers: the lower mantle, which is extremely viscous, then a layer of lower viscosity above it, and then a layer of increased viscosity above that which is the upper mantle.
Aside from computer modelling, what evidence exists that slap orphaning actually happens?
One of the places where we think orphaning is happening is at the Tonga Trench (a deep oceanic trench in the southwestern Pacific Ocean). If you look at seismic images of the subducting slab there is a toe at the end of the Tonga slab that is lying flat on top of the lower mantle with another smaller slab lying directly underneath it.
We can test our hypothesis through seismic tomography (a computer-enhanced interpretation of seismic data) and indeed we do see slab orphaning at several subduction zones on Earth. Higher resolution seismic tomography will give us better insights, in fact we are currently collaborating with a seismologist who has done some very high resolution seismic imaging of Pacific slabs where we can clearly see a slab that is in the process of orphaning. This is a very good indication that slab orphaning has happened in the past and is still happening today.
Does this change our understanding of Earth processes like earthquakes?
It’s not going to help us understand when the next big earthquake is going to happen, but it helps us understand how slabs behave at depth. If we can understand how deep slabs behave, we can better understand how this translates to the surface, and what that means in terms of surface deformation. And surface deformation in practical terms does mean earthquakes. So if we understand the behavior of slabs, we can understand better their relation to the surface, and that means that we can understand how earthquakes are related to subduction zones, and how that then relates to hazard mitigation.
The biggest change is in our understanding of what these deep fragments are and how they got there. Previous work used to look at these deep fragments and assume that they are remnants of older subductions that are now extinct — a blob of old slab material that broke much higher up and then slowly sank over millions and millions of years under its own weight through the mantle.
Whereas with orphaning, the slab fragment was carried there as part of the bigger slab and then broke in the last 5 million years (as opposed to the last 200 million years). So, I think orphaning helps people think about the link between these fragments and the timing of what’s going on at the surface. That’s important because it can help us more accurately recreate what our planet’s geography and climate was like in the distant past.
What’s next in this line of research?
I’m working on a very exciting project at UTIG to understand how subducted slabs in the mantle can transmit stresses to the surface and build mountains or even break up the overriding plate to initiate new subduction. One of the areas I am studying is Colombia, specifically how the flat slab that is subducting underneath Colombia is shaping the deformation and uplift of the continental overriding plate.
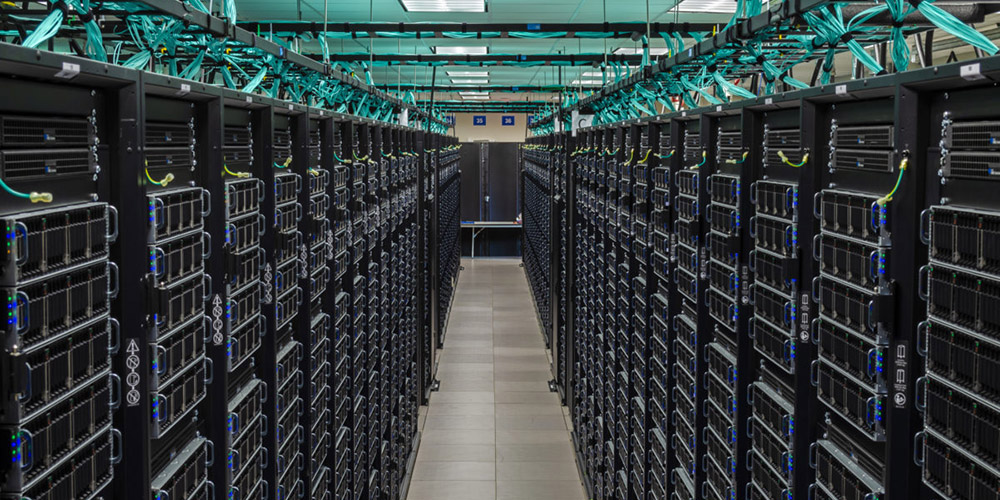
I’ve also had a proposal accepted to run mantle convection models using TACC’s Frontera supercomputer, which I’ll be doing with (Jackson School grad students) Wanying Wang and Erin Heilman. Having access to the world’s most powerful academic supercomputer means that for the first time we are able to run super high resolution (at the scale of a few kilometers) global convection models to help us understand the physics of plate boundary evolution, subduction dynamics and tectonic surface deformation. These will shed light on the earthquake cycle, deep slab behavior, the evolution of topography and help us understand why Earth is the only planet with long-lived plate tectonics.
I have also received UTIG Blue Skies funding together with Professor (Thorsten) Becker and Dr. (Krista) Soderlund, to explore subduction evolution on the icy surface of Europa and the early Earth. Europa is the only place in our solar system other than Earth to exhibit clear signs of active plate tectonics-like behavior, which we believe are similar to those that shaped the Earth 3.7 billion years ago. The plan is to unlock the physics of ice-subduction, and understand how these intermittent, shallow depth processes evolved to modern-day Earth plate tectonics that can sustain life and support a habitable planet.